It is evident that the ceiling for the new energy sector is higher than anticipated, with capital still pouring in, seemingly in search of the next "Contemporary Amperex Technology" or "BYD."
Overview
Sodium-ion batteries (referred to as "sodium batteries") are a type of rechargeable battery that operates by shuttling sodium ions between the cathode and anode during charging and discharging. Their working principle and structure are similar to the widely used lithium-ion batteries.
Both sodium and lithium belong to the same group of elements and exhibit similar "rocking chair" electrochemical charging and discharging behaviors. During the charging process of a sodium-ion battery, sodium ions detach from the cathode and embed into the anode while electrons travel through the external circuit. The more sodium ions embedded in the anode, the higher the charging capacity. Conversely, during discharging, sodium ions return from the anode to the cathode, increasing the discharge capacity as more sodium ions move back.
Working Principle
The working principle of sodium-ion batteries is akin to that of lithium-ion batteries, involving the insertion and extraction of sodium ions to achieve charge transfer. During discharge, sodium ions exit the anode material and enter the cathode material, with electrons flowing from the anode to the cathode, releasing energy.
During charging, sodium ions detach from the cathode material and move into the anode material through the electrolyte, while electrons flow into the anode material through the external circuit. Ideally, the insertion and extraction of ions during charging and discharging should not alter the material's structure or cause side reactions with the electrolyte. However, current technology faces challenges due to the larger radius of sodium ions, which leads to material structure changes during ion insertion, resulting in decreased cycle performance and stability.
Advantages
Energy Density: Sodium-ion battery cells typically have an energy density of 100-150 Wh/kg, while lithium-ion battery cells generally range from 120-200 Wh/kg, with high-nickel ternary systems exceeding 200 Wh/kg. Although sodium-ion batteries currently have a lower energy density compared to ternary lithium batteries, they can partially overlap or cover the energy density range of lithium iron phosphate batteries (120-200 Wh/kg) and lead-acid batteries (30-50 Wh/kg).
Operating Temperature Range and Safety: Sodium-ion batteries operate over a wide temperature range, typically from -40°C to 80°C. In contrast, ternary lithium-ion batteries usually operate between -20°C and 60°C, with performance declining below 0°C. Sodium-ion batteries can maintain over 80% State of Charge (SOC) at -20°C. Additionally, due to higher internal resistance, sodium-ion batteries are less prone to heating during short circuits, offering greater safety compared to lithium-ion batteries.
Rate Performance: The charge and discharge rate performance of sodium-ion batteries is directly related to the migration ability of sodium ions at the electrode-electrolyte interface. Factors affecting ion migration speed impact the battery's rate performance. Additionally, the internal heat dissipation rate is crucial for safety and lifespan during high-rate charging and discharging. Thanks to their crystal structure, sodium-ion batteries exhibit good rate performance, making them suitable for energy storage and large-scale power supply applications.
Charging Speed: Sodium-ion batteries can be fully charged in about 10 minutes, whereas ternary lithium batteries require at least 40 minutes, and lithium iron phosphate batteries need around 45 minutes.
Industry Classification
Sodium-ion batteries come in various types, including sodium-sulfur batteries, sodium-salt batteries, sodium-air batteries, aqueous sodium-ion batteries, organic sodium-ion batteries, and solid-state sodium-ion batteries.
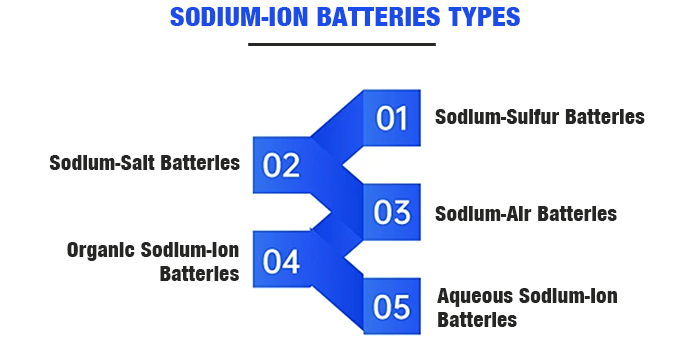
In the energy storage sector, the primary commercially applied sodium batteries include high-temperature sodium-sulfur batteries and sodium-metal chloride batteries based on solid electrolyte systems. These systems use metallic sodium as the active anode material, more accurately termed sodium batteries. Typically, the term sodium-ion battery refers to the latter three types.
Sodium-Sulfur Batteries: These use molten liquid sodium as the anode and elemental sulfur as the cathode, with solid ceramic Al2O3 as the electrolyte and separator. Sodium-sulfur batteries have a high specific energy.
Sodium-Salt Batteries: These use liquid sodium as the anode and metal chloride materials as the cathode, with Na+ conductor Al2O3 ceramic as the electrolyte.
Sodium-Air Batteries: The cathode typically uses porous materials, which provide pathways for gas diffusion and sites for electrode reactions due to the material's porosity.
Organic Sodium-Ion Batteries: These use hard carbon or sodium-intercalated materials for the anode, with cathode materials including transition metal oxides and polyanionic compounds.
Aqueous Sodium-Ion Batteries: Compared to organic electrolyte batteries, aqueous sodium-ion batteries use different electrolytes, offering higher safety performance.