With technological advancements and industry scaling, the cost of photovoltaic (PV) power generation continues to decrease, positioning it as a pivotal energy source for sustainable development in the future.
Key Components of Photovoltaic Technology
The core component of PV power generation technology is the solar PV cell. The evolution of solar PV cells can be categorized into three generations. The first generation consists of silicon-based solar cells; the second generation includes thin-film solar cells; and the third generation encompasses new technologies such as high-concentration photovoltaic (HCPV) cells, organic solar cells, flexible solar cells, and dye-sensitized solar cells. Currently, silicon-based solar cells dominate the market, while thin-film cells are gradually gaining market share. Most third-generation cells, except for HCPV, are still in the research phase.
Silicon-Based Solar Cells
Among silicon-based solar cells, monocrystalline silicon technology is the most mature. The efficiency and cost of these cells are primarily influenced by the manufacturing process, which includes steps such as ingot casting, wafer slicing, diffusion, texturing, screen printing, and sintering. Solar cells produced through this conventional process typically achieve a photoelectric conversion efficiency of 16-18%.
Monocrystalline silicon solar cells have the highest conversion efficiency but are also the most expensive. Polycrystalline silicon solar cells offer a good cost reduction by directly manufacturing large-size square silicon ingots suitable for mass production. This process is simpler, saves power, conserves silicon material, and requires lower material quality.
Reducing the cost of solar cells can be achieved through two main strategies: decreasing material consumption (e.g., reducing silicon wafer thickness) and increasing conversion efficiency. Methods to enhance efficiency include increasing light absorption (e.g., surface texturing, anti-reflective coating, reducing front electrode width), reducing recombination of photogenerated carriers (e.g., emitter passivation), and minimizing resistance (e.g., localized doping, back surface field technology).
The highest recorded conversion efficiency for monocrystalline silicon solar cells is 24.7%, achieved by the PERL structure solar cell from the University of New South Wales. Key technological features include low phosphorus doping concentration on the silicon surface to reduce surface recombination, high-concentration diffusion under front and rear surface electrodes to form good ohmic contacts, and the use of photolithography to narrow the front surface electrodes, increasing the light absorption area. However, this technology has yet to be industrialized.
Other techniques to improve efficiency include BP Solar's surface grooved textured cells and back-contact (EWT) technology. The former achieves an efficiency of 18.3% through laser grooving, which reduces the width of the front electrodes and increases light absorption. The latter achieves an efficiency of 21.3% by bringing the front electrodes to the back, increasing the light-absorbing area.
Thin-Film Solar Cells
While crystalline silicon solar cells dominate due to their high efficiency, reducing their cost significantly is challenging due to the high price of silicon material. Thin-film solar cells, which use less material, have emerged as a cost-effective alternative. The main types of thin-film cells include silicon-based thin-film cells, cadmium telluride (CdTe) cells, and copper indium gallium selenide (CIGS) cells.
Silicon-based thin-film cells are only 2 micrometers thick, using about 1.5% of the silicon material required for crystalline silicon cells. Depending on the number of PN junctions, these cells can be single-junction, double-junction, or multi-junction, each capable of absorbing different wavelengths of sunlight. The highest efficiency for single-junction cells is around 7%, while double-junction cells can reach 10%.
CdTe thin-film cells offer higher efficiency (up to 12%) due to their good light absorption properties. However, the carcinogenic nature of cadmium and the limited natural reserves of tellurium pose long-term development challenges.
CIGS thin-film cells are considered the future of high-efficiency thin-film technology. By adjusting the manufacturing process, their light absorption can be improved, leading to higher conversion efficiencies. Currently, laboratory efficiencies reach 20.1%, while commercial products achieve 13-14%, making them the most efficient among thin-film cells.
Third-Generation Cells
Theoretically, third-generation cells can achieve high conversion efficiencies. Except for HCPV, most are still in the research stage. HCPV cells typically use III-V semiconductor materials, which have higher heat resistance and maintain high conversion efficiency under high illumination. Multi-junction structures allow these cells to match the solar spectrum closely, with theoretical efficiencies up to 68%. Commercial production can achieve efficiencies above 40%.
Solar cells are encapsulated into modules, and their applications depend on their characteristics and market demands. Early applications included communication base stations and satellites, later expanding to residential areas like solar rooftops. In these scenarios, limited installation areas and high energy density needs favored crystalline silicon modules. With the development of large-scale solar power plants and building-integrated photovoltaics (BIPV), cost considerations have led to increased thin-film cell applications. Environmental and climatic conditions also influence the adoption of different technologies.
Applications of Solar Photovoltaic Technology
Converting solar radiation into usable electricity requires a complete solar PV system. Solar PV cells form the foundation of this system, which also includes inverters, batteries, monitoring systems, and distribution systems.
PV System Classification and Composition
Solar PV systems are classified as either off-grid or grid-tied. Off-grid systems can be standalone or hybrid.
Standalone systems are typically used in remote areas, communication base stations, and solar streetlights, relying entirely on solar energy. They include solar modules, inverters, controllers, batteries, distribution systems, and lightning protection. Batteries and controllers significantly impact system cost and lifespan. Hybrid systems combine solar energy with other sources like diesel generators or wind turbines.
Grid-tied systems, commonly used for solar rooftops and large-scale PV power plants, do not require storage equipment, reducing costs. These systems include solar modules, inverters, distribution systems, lightning protection, and monitoring systems. Currently, grid-tied systems account for 80% of all solar applications.
Other PV Power Generation Technologies
Besides solar PV cell technology, inverter technology, grid integration, storage, and intelligent monitoring are crucial for PV power generation systems:
Solar cell output power varies with solar radiation intensity, causing intermittency. Large-scale grid integration can impact the grid, making grid control and islanding protection essential.
Solar module output is direct current (DC), requiring high-quality conversion to alternating current (AC) via inverters.
Module power output can be affected by factors like temperature and shading, necessitating system monitoring and alarm systems.
Remote control technology is vital for PV power plants in remote areas.
China leads in solar module production in terms of quality and scale. High-profit areas within the industry chain include silicon purification, inverters, monitoring systems, and PV equipment manufacturing. Achieving breakthroughs in these key areas is a challenge for China's PV industry.
Current Status and Future Prospects of Solar PV Power Generation
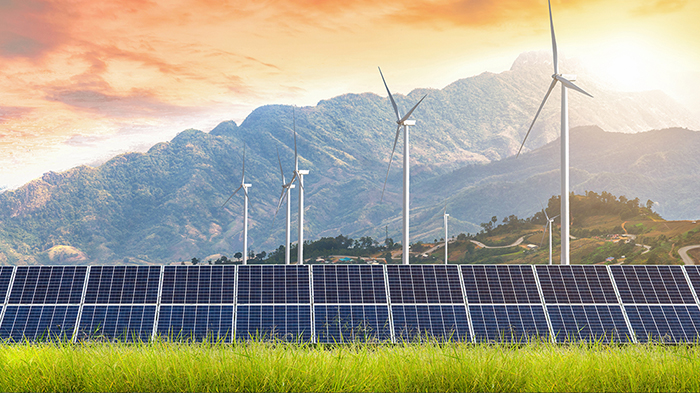
Due to high costs, solar PV power generation did not see large-scale development until the end of the last century. Entering the 21st century, with improved efficiency and rapidly decreasing costs, solar PV power generation has experienced rapid growth, with installed capacity increasing annually. Global annual installed capacity rose from 1.4 GW in 2000 to 22.8 GW in 2009. European countries like Germany, Italy, and Spain are major markets, with the EU planning to increase the share of solar power to 12% of total electricity supply by 2020. Developing countries like China and India have also launched solar development plans. Beyond communication base stations, solar rooftops, and PV power plants, solar PV power generation is now widely used in various mobile devices.
As a supplementary and alternative energy source, solar PV technology is developing rapidly, with decreasing generation costs. With ongoing technological advancements, solar energy, as a clean and renewable resource, is poised to become a key energy source for sustainable development.